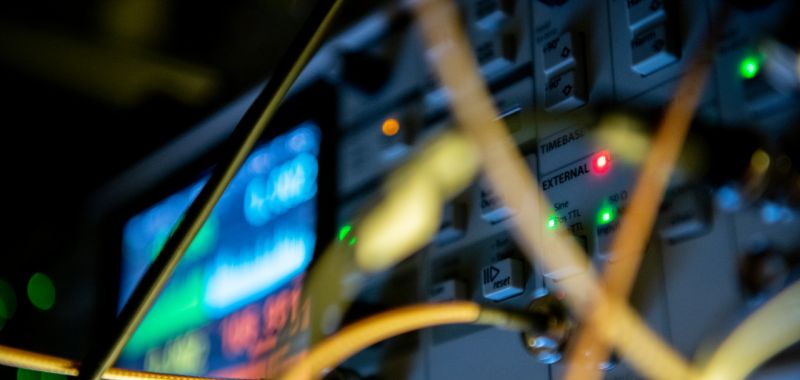
Benchmarking DFT functionals for non-radiative decay
Exciton Science researchers have developed the first benchmarking study of density functionals for use in mapping non-radiative decay pathways between excited states.
Non-radiative decay pathways play a significant role in the excited state dynamics of photoactive molecules, which in turn impact the efficiencies of photon-harvesting devices, including solar cells.
Many of these pathways are well understood and can be predicted in their speed using theoretical models.
Same-spin (or nonadiabatic) pathways, however, are particularly difficult to model due to the complexity of scaling with respect to the system size.
DFT has been successful in calculating nonadiabatic matrix coupling elements and internal conversion rates within excited states before.
However, the applicability and accuracy of various density functionals towards same-spin non-radiative rates, as well as the exchange–correlation component of the functional, remain unknown.
This work used a monomer perylene chromophore as a case study, and comprehensively surveyed the accuracy of density functionals for calculating non-radiative decay pathways.
The researchers, based across RMIT University and the University of Melbourne, were able to identify several treatments within density functional theory which can reproduce experimental data to a high degree of fidelity.
The results have been published in the Journal of Chemical Theory and Computation and are available here.
First author Dr Anjay Manian of RMIT University said: “The work looks at a sample system and goes through every point that you would use the theoretical approach.
“It starts completely from first principles, goes from the geometry, builds up the energies, the couplings, and then actually starts calculating the photoluminescence quantum yield, which is a very important parameter for things like photon harvesting applications.”
The next step is to take these methods and apply this knowledge to better understand similar processes from high-lying molecular excited states.